Secondary Structure of Deoxyribonucleic Acids:
Some observations made on deoxyribonucleic acids in solution suggested that DNA molecules — as they could be extracted from cells — had structural characteristics different from those expected in molecules having only the primary structure we just described; on the contrary, after heating, the DNA molecules behaved like simple flexible chains.
It therefore appeared that native DNA has a secondary structure and it must be said to the credit of Crick and Watson that they proposed in 1953, a model which explained the various properties of the DNA.
Crick and Watson Established their Model on the Basis of Some Facts:
i. Results of base analyses which showed in particular, that there is as much adenine as thymine and as much guanine as cytosine in deoxyribonucleic acids (A/T and G/C = 1). Therefore, the sum of purines is equal to the sum of pyrimidines (A + G = C + T). On the contrary, the ratio A + T/G + C varies considerably from one DNA to another; it is characteristic of the species, and is called the dissymmetry ratio (if it is greater than 1, the DNA is rich in A-T, if it is less than 1 the DNA is rich in G-C);
ii. Results of X-ray diffraction studies (carried out by Wilkins) which suggested a helicoidal configuration;
iii. Experimental results which suggested that the polydeoxyribonucleotide chains were held together by hydrogen bonds.
Let us describe the important characteristics of this model illustrated in figure 6-15.
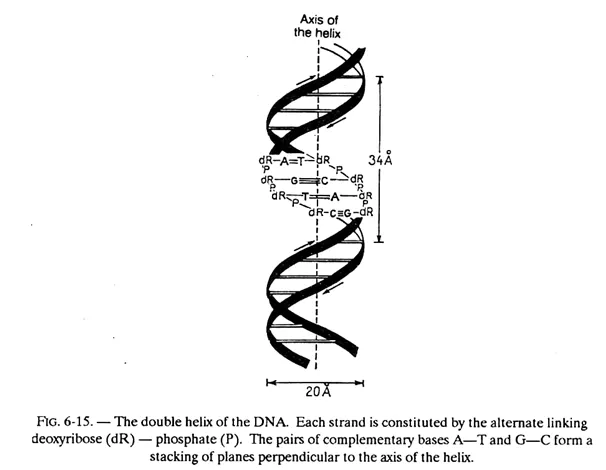
The DNA molecule consists of two polydeoxyribonucleotide chains twisted around one another in a double helix.
The bases of each of these chains are projected towards the centre (i.e. towards the axis of the helix); the planes formed by the atoms of these bases are parallel to one another and perpendicular to the axis of the double helix. These planes are 3.4 Å apart and since the pitch of the helix is 34 Å, there will be 10 planes per turn.
In fact, these planes consist of, not one base but one base of each chain (i.e. one pair of bases) held together by hydrogen bonds. While, in principle any base when free, can exchange one or several hydrogen bonds with any other base, the configuration of the helix is such that a stable pairing — obtained by the exchange of more than one hydrogen bond — can take place only between adenine and thymine on the one hand (2 hydrogen bonds) and between guanine and cytosine on the other (3 hydrogen bonds). Adenine and thymine are said to be complementary bases, as also guanine and cytosine.
One now understands why the A/T and G/C ratios are equal to 1; as a matter of fact, to one adenine in a chain, must necessarily correspond one thymine in the other chain (and conversely), and to one guanine in one chain must necessarily correspond one cytosine in the other chain (and conversely).
Hence, the base sequence in one of the chains automatically determines the sequence in the other chain and we will see that this is of great importance in the study of the replication of the DNA.
The 2 chains (or strands) of a DNA molecule are complementary (not identical). Besides, they are antiparallel, as may be observed in figure 6-16; in other words they “run” in opposite direction (their 3′-5′ phosphodiester bonds are oriented in opposite directions); this can be demonstrated and we shall revert to this aspect in fig. 6-30.
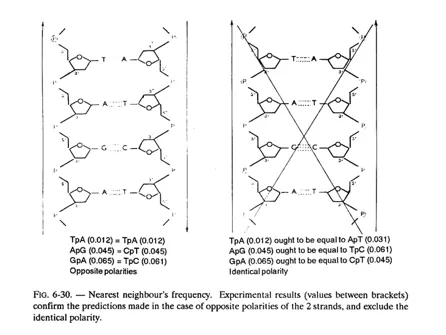
The hydrogen bonds between complementary bases are partly responsible for holding the 2 chains in a helicoidal configuration, but some other forces are also involved, especially the hydrophobic and electronic interactions between the bases stacked on one another.
This double-stranded helicoidal configuration is a general characteristic of deoxyribonucleic acids; the Crick and Watson model is thus valid for the deoxyribonucleic acids of bacteriophages, bacteria, plants and animals. There are however some rare exceptions, particularly the DNA of the bacteriophage ϕ X 1744, isolated by Sinsheimer in 1959, which has a single-stranded structure (however, during the infection of E.coli cells, the double-stranded form, called replicative form, appears in the host bacterium).
The DNAs can in fact, adopt several three-dimensional conformations and change reversibly from one form to the other.
The B form of the helix is characterized by the presence of a large groove (width 12 Å, depth 8.5 Å) and a small groove (width 6 Å, depth 7.5 Å), because the glycosidic bonds of a base pair are not diametrically opposed. The capacities of these 2 grooves in respect of the donor and acceptor atoms of hydrogen bonds are not the same.
The large groove is more accessible to proteins interacting with specific sequences of the DNA. We have seen in the foregoing that, according to the Crick and Watson model, the double helix comprises 10 base pairs per turn, such that from one residue to the following on the chain, there is a rotation of 36°, but in reality this rotation angle can vary
from 28° to 42°.
Furthermore, the 2 bases of a pair are not absolutely coplanar, they are turned with respect to one another like the blades of an aircraft propeller which increases the stacking of bases in each strand. Also, the base pairs are more or less inclined with respect to the neighbouring pairs.
All these localized variations of the structure of the double helix depend on the nucleotide sequence, and can be recognized by a protein in search of its target region on the DNA. Lastly, the B helix can be deformed (curved, folded back, or twisted to form a super-helix), which permits for example the formation of covalent circles or the twisting of the DNA around proteins (for example, to form the nucleosomes).
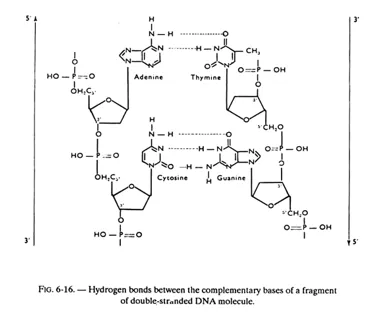
Form A is, like form B, a double helix twisted on its right, but it is wider and the base pairs are inclined at 19° instead of being perpendicular to the axis of the helix. These differences are due to the fact that the carbon atom in 3′ of the furanose ring is at 0.5Å from the plane formed by the 4 other atoms of pentose (C — 3′ endo). The phosphate groups can bind less water molecules than in the form B, and the small groove is practically non-existent.
Form Z is on the contrary, a double helix turning to its left. It owes its name to the fact that the phosphates of the chain form a zigzag. Form Z has only one deep groove.
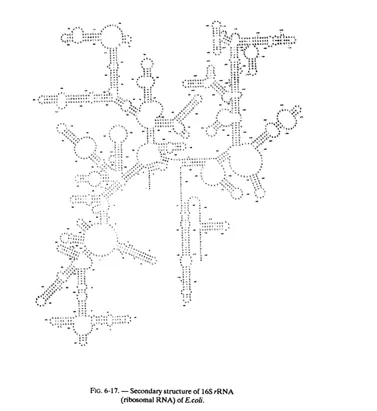
The existence of form Z is facilitated by an alternance of purines and pyrimidines (as for example in the sequence CGCGCG), which permits at the glycosidic bonds, an alternance of conformations syn (base and sugar are near one another, on the same side) and anti (base and sugar are distant, because they are on opposite sides).
While in forms A and B of the DNA all glycosidic bonds are anti, in form Z the purines are syn and the pyrimidines are anti. Although the major part of the DNA is in form B, in procaryotes as well as in eucaryotes, the specific antibodies of form Z enable one to detect regions of DNA-Z in the chromosomes of eucaryotes.
The formation of DNA-Z is facilitated by the methylation of C-5 of cytosine (a modification observed in eucaryotes and which favours hydrophobic interactions) and by the negative supercoiling of native molecules of DNA (which promotes the separation of the 2 strands of the helix in view of replication and transcription).
Secondary Structure of Ribonucleic Acids:
Hydrogen bonds can also be formed between adenine and uracil on the one hand and guanine and cytosine on the other. But first, these bonds are formed between 2 bases of the same chain (whereas in the DNA the hydrogen bonds are interchain), and moreover these bonds do not present the regularity characteristic of deoxyribonucleic acids (where the complementary of bases is total, i.e. the bases are paired all along the molecule).
However, after such pairing, the RNA chains can take in some zones, a helicoidal configuration, sometimes called pseudo-helicoidal .
But it must be mentioned that there are double-stranded replicative forms of some viral ribonucleic acids which have a regular double helix structure similar to that of deoxyribonucleic acids; actually, the single-stranded RNA which constitutes the genome of the virus serves, during the infection, as template for the synthesis of a complementary strand and double-stranded structures are then observed transitorily.