Genotype versus Phenotype
All cellular activities are encoded within a cell’s DNA. The sequence of bases within a DNA molecule represents the genetic information of the cell. Segments of DNA molecules are called genes, and individual genes contain the instructional code necessary for synthesizing various proteins, enzymes, or stable RNA molecules.
The full collection of genes that a cell contains within its genome is called its genotype. However, a cell does not express all of its genes simultaneously. Instead, it turns on (expresses) or turns off certain genes when necessary. The set of genes being expressed at any given point in time determines the cell’s activities and its observable characteristics, referred to as its phenotype. Genes that are always expressed are known as constitutive genes; some constitutive genes are known as housekeeping genes because they are necessary for the basic functions of the cell.
While the genotype of a cell remains constant, the phenotype may change in response to environmental signals (e.g., changes in temperature or nutrient availability) that affect which nonconstitutive genes are expressed. For example, the oral bacterium Streptococcus mutans produces a sticky slime layer that allows it to adhere to teeth, forming dental plaque; however, the genes that control the production of the slime layer are only expressed in the presence of sucrose (table sugar). Thus, while the genotype of S. mutans is constant, its phenotype changes depending on the presence and absence of sugar in its environment. Temperature can also regulate gene expression. For example, the gram-negative bacterium Serratia marcescens, a pathogen frequently associated with hospital-acquired infections, produces a red pigment at 28 °C but not at 37 °C, the normal internal temperature of the human body (Figure 1).
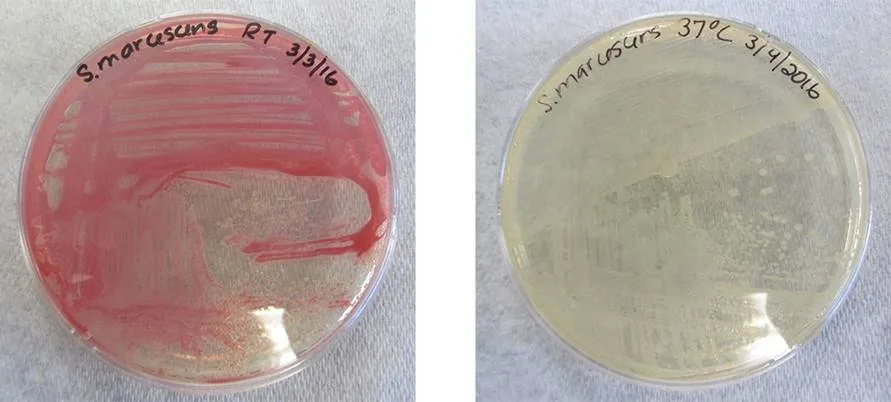
Figure 1. Both plates contain strains of Serratia marcescens that have the gene for red pigment. However, this gene is expressed at 28 °C (left) but not at 37 °C (right).
Organization of Genetic Material
The vast majority of an organism’s genome is organized into the cell’s chromosomes, which are discrete DNA structures within cells that control cellular activity. Recall that while eukaryotic chromosomes are housed in the membrane-bound nucleus, most prokaryotes contain a single, circular chromosome that is found in an area of the cytoplasm called the nucleoid (see Unique Characteristics of Prokaryotic Cells). A chromosome may contain several thousand genes.
Organization of Eukaryotic Chromosome
Chromosome structure differs somewhat between eukaryotic and prokaryotic cells. Eukaryotic chromosomes are typically linear, and eukaryotic cells contain multiple distinct chromosomes. Many eukaryotic cells contain two copies of each chromosome and, therefore, are diploid.
The length of a chromosome greatly exceeds the length of the cell, so a chromosome needs to be packaged into a very small space to fit within the cell. For example, the combined length of all of the 3 billion base pairsof DNA of the human genome would measure approximately 2 meters if completely stretched out, and some eukaryotic genomes are many times larger than the human genome. DNA supercoiling refers to the process by which DNA is twisted to fit inside the cell. Supercoiling may result in DNA that is either underwound (less than one turn of the helix per 10 base pairs) or overwound (more than one turn per 10 base pairs) from its normal relaxed state. Proteins known to be involved in supercoiling include topoisomerases; these enzymes help maintain the structure of supercoiled chromosomes, preventing overwinding of DNA during certain cellular processes like DNA replication.
During DNA packaging, DNA-binding proteins called histones perform various levels of DNA wrapping and attachment to scaffolding proteins. The combination of DNA with these attached proteins is referred to as chromatin. In eukaryotes, the packaging of DNA by histones may be influenced by environmental factors that affect the presence of methyl groups on certain cytosine nucleotides of DNA. The influence of environmental factors on DNA packaging is called epigenetics. Epigenetics is another mechanism for regulating gene expression without altering the sequence of nucleotides. Epigenetic changes can be maintained through multiple rounds of cell division and, therefore, can be heritable.
Genotype versus Phenotype
All cellular activities are encoded within a cell’s DNA. The sequence of bases within a DNA molecule represents the genetic information of the cell. Segments of DNA molecules are called genes, and individual genes contain the instructional code necessary for synthesizing various proteins, enzymes, or stable RNA molecules.
The full collection of genes that a cell contains within its genome is called its genotype. However, a cell does not express all of its genes simultaneously. Instead, it turns on (expresses) or turns off certain genes when necessary. The set of genes being expressed at any given point in time determines the cell’s activities and its observable characteristics, referred to as its phenotype. Genes that are always expressed are known as constitutive genes; some constitutive genes are known as housekeeping genes because they are necessary for the basic functions of the cell.
While the genotype of a cell remains constant, the phenotype may change in response to environmental signals (e.g., changes in temperature or nutrient availability) that affect which nonconstitutive genes are expressed. For example, the oral bacterium Streptococcus mutans produces a sticky slime layer that allows it to adhere to teeth, forming dental plaque; however, the genes that control the production of the slime layer are only expressed in the presence of sucrose (table sugar). Thus, while the genotype of S. mutans is constant, its phenotype changes depending on the presence and absence of sugar in its environment. Temperature can also regulate gene expression. For example, the gram-negative bacterium Serratia marcescens, a pathogen frequently associated with hospital-acquired infections, produces a red pigment at 28 °C but not at 37 °C, the normal internal temperature of the human body (Figure 1).
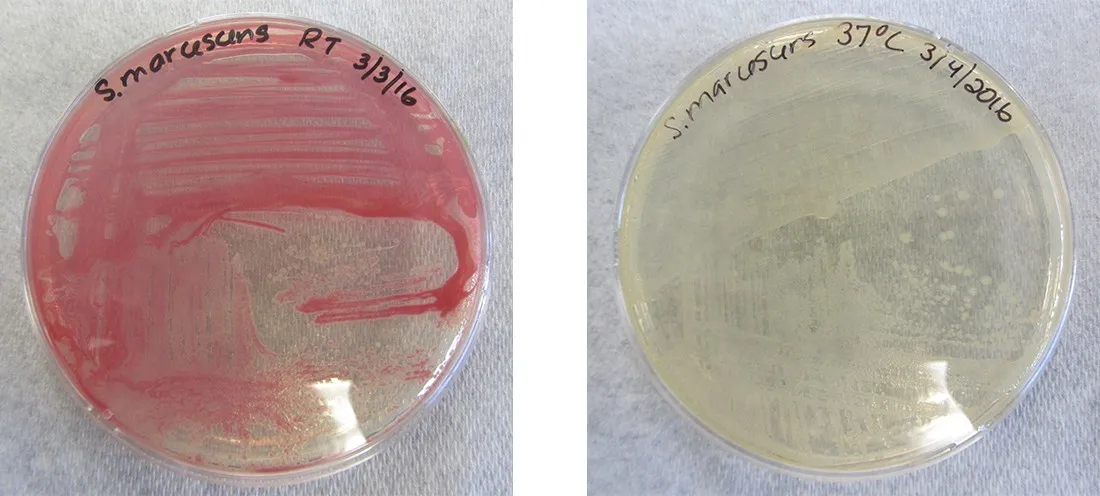
Figure 1. Both plates contain strains of Serratia marcescens that have the gene for red pigment. However, this gene is expressed at 28 °C (left) but not at 37 °C (right).
Organization of Genetic Material
The vast majority of an organism’s genome is organized into the cell’s chromosomes, which are discrete DNA structures within cells that control cellular activity. Recall that while eukaryotic chromosomes are housed in the membrane-bound nucleus, most prokaryotes contain a single, circular chromosome that is found in an area of the cytoplasm called the nucleoid (see Unique Characteristics of Prokaryotic Cells). A chromosome may contain several thousand genes.
Organization of Eukaryotic Chromosome
Chromosome structure differs somewhat between eukaryotic and prokaryotic cells. Eukaryotic chromosomes are typically linear, and eukaryotic cells contain multiple distinct chromosomes. Many eukaryotic cells contain two copies of each chromosome and, therefore, are diploid.
The length of a chromosome greatly exceeds the length of the cell, so a chromosome needs to be packaged into a very small space to fit within the cell. For example, the combined length of all of the 3 billion base pairs[1] of DNA of the human genome would measure approximately 2 meters if completely stretched out, and some eukaryotic genomes are many times larger than the human genome. DNA supercoiling refers to the process by which DNA is twisted to fit inside the cell. Supercoiling may result in DNA that is either underwound (less than one turn of the helix per 10 base pairs) or overwound (more than one turn per 10 base pairs) from its normal relaxed state. Proteins known to be involved in supercoiling include topoisomerases; these enzymes help maintain the structure of supercoiled chromosomes, preventing overwinding of DNA during certain cellular processes like DNA replication.
During DNA packaging, DNA-binding proteins called histones perform various levels of DNA wrapping and attachment to scaffolding proteins. The combination of DNA with these attached proteins is referred to as chromatin. In eukaryotes, the packaging of DNA by histones may be influenced by environmental factors that affect the presence of methyl groups on certain cytosine nucleotides of DNA. The influence of environmental factors on DNA packaging is called epigenetics. Epigenetics is another mechanism for regulating gene expression without altering the sequence of nucleotides. Epigenetic changes can be maintained through multiple rounds of cell division and, therefore, can be heritable.
Organization of Prokaryotic Chromosomes
Chromosomes in bacteria and archaea are usually circular, and a prokaryotic cell typically contains only a single chromosome within the nucleoid. Because the chromosome contains only one copy of each gene, prokaryotes are haploid. As in eukaryotic cells, DNA supercoiling is necessary for the genome to fit within the prokaryotic cell. The DNA in the bacterial chromosome is arranged in several supercoiled domains. As with eukaryotes, topoisomerases are involved in supercoiling DNA. DNA gyrase is a type of topoisomerase, found in bacteria and some archaea, that helps prevent the overwinding of DNA. (Some antibiotics kill bacteria by targeting DNA gyrase.) In addition, histone-like proteins bind DNA and aid in DNA packaging. Other proteins bind to the origin of replication, the location in the chromosome where DNA replication initiates. Because different regions of DNA are packaged differently, some regions of chromosomal DNA are more accessible to enzymes and thus may be used more readily as templates for gene expression. Interestingly, several bacteria, including Helicobacter pylori and Shigella flexneri, have been shown to induce epigenetic changes in their hosts upon infection, leading to chromatin remodeling that may cause long-term effects on host immunity.
Noncoding DNA
In addition to genes, a genome also contains many regions of noncoding DNA that do not encode proteins or stable RNA products. Noncoding DNA is commonly found in areas prior to the start of coding sequences of genes as well as in intergenic regions (i.e., DNA sequences located between genes) (Figure 2).
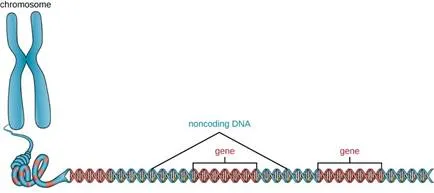
Figure 2. Chromosomes typically have a significant amount of noncoding DNA, often found in intergenic regions.
Prokaryotes appear to use their genomes very efficiently, with only an average of 12% of the genome being taken up by noncoding sequences. In contrast, noncoding DNA can represent about 98% of the genome in eukaryotes, as seen in humans, but the percentage of noncoding DNA varies between species.[3] These noncoding DNA regions were once referred to as “junk DNA”; however, this terminology is no longer widely accepted because scientists have since found roles for some of these regions, many of which contribute to the regulation of transcription or translation through the production of small noncoding RNA molecules, DNA packaging, and chromosomal stability. Although scientists may not fully understand the roles of all noncoding regions of DNA, it is generally believed that they do have purposes within the cell.
Extrachromosomal DNA
Although most DNA is contained within a cell’s chromosomes, many cells have additional molecules of DNA outside the chromosomes, called extrachromosomal DNA, that are also part of its genome. The genomes of eukaryotic cells would also include the chromosomes from any organelles such as mitochondria and/or chloroplasts that these cells maintain (Figure 3). The maintenance of circular chromosomes in these organelles is a vestige of their prokaryotic origins and supports the endosymbiotic theory (see Foundations of Modern Cell Theory). In some cases, genomes of certain DNA viruses can also be maintained independently in host cells during latent viral infection. In these cases, these viruses are another form of extrachromosomal DNA. For example, the human papillomavirus (HPV) may be maintained in infected cells in this way.
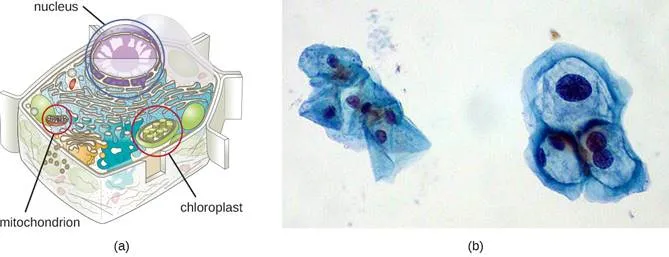
Figure 3. The genome of a eukaryotic cell consists of the chromosome housed in the nucleus, and extrachromosomal DNA found in the mitochondria (all cells) and chloroplasts (plants and algae).
Besides chromosomes, some prokaryotes also have smaller loops of DNA called plasmids that may contain one or a few genes not essential for normal growth (see Figure 1 in Unique Characteristics of Prokaryotic Cells). Bacteria can exchange these plasmids with other bacteria in a process known as horizontal gene transfer (HGT). The exchange of genetic material on plasmids sometimes provides microbes with new genes beneficial for growth and survival under special conditions. In some cases, genes obtained from plasmids may have clinical implications, encoding virulence factors that give a microbe the ability to cause disease or make a microbe resistant to certain antibiotics. Plasmids are also used heavily in genetic engineering and biotechnology as a way to move genes from one cell to another. The role of plasmids in horizontal gene transfer and biotechnology will be discussed further in Mechanisms of Microbial Genetics and Modern Applications of Microbial Genetics.
Viral Genomes
Viral genomes exhibit significant diversity in structure. Some viruses have genomes that consist of DNA as their genetic material. This DNA may be single stranded, as exemplified by human parvoviruses, or double stranded, as seen in the herpesviruses and poxviruses. Additionally, although all cellular life uses DNA as its genetic material, some viral genomes are made of either single-stranded or double-stranded RNA molecules, as we have discussed. Viral genomes are typically smaller than most bacterial genomes, encoding only a few genes, because they rely on their hosts to carry out many of the functions required for their replication. The diversity of viral genome structures and their implications for viral replication life cycles are discussed in more detail in The Viral Life Cycle.