Introduction
In the beginning, there was biology. It reigned supreme over the other subject areas in splendor and elegance. It defined and explained the living parts of the world and their environment. What else is there to know?
An understanding of biology requires a little knowledge of chemistry, and an understanding of chemistry requires a little knowledge of mathematics—that’s where we draw the line. It is important to know aspects of chemistry to make biology come alive, but it is not important to go into detailed mathematical applications to understand the principles of biology—so we won’t!
Modern biology overlaps with chemistry in explaining the structure and function of all cellular processes at the molecular level. Several important chemical concepts are treated in detail in the sections that follow. When applied in later sections, these chemical concepts will allow you to construct greater meaning of the more complex biological principles.
Atomic Theory
For biological purposes, the simplest form of a pure substance that retains all of the properties of that substance is an atom. Atoms are also the simplest form of an element. An element is made up of only one type of atom. For instance, carbon is an element. All carbon atoms look the same and they are the only atoms that are carbon atoms. A different atomic structure would be a different atom and therefore a different element. Consult a periodic table for the complete listing of the 100+ elements (for example, pearl1.lanl.gov/periodic/default.htm). All atoms have three components: protons, neutrons, and electrons. The first element, hydrogen, has one proton, usually one neutron, and one electron. (Hydrogen exists in nature as a combination of three isotopes each of which has a different number of neutrons. Normally the most prominent isotope which has one proton is considered.) All hydrogen atoms for the most part look the same.
Protons are a positive charge and along with neutrons, which carry no charge (neutral), are found in the centrally located nucleus of the atom. Electrons are negatively charged and orbit around the nucleus in a three-dimensional cloud of energy levels. The positive-negative attraction (magnetism) keeps the electrons from flying away from the nucleus. Conversely, the kinetic energy (energy associated with motion) of the electron provides it with the energy of movement and keeps the electron from slamming into the nucleus. The balance between these two forces, magnetism and kinetic energy, defines the orbital pathways of all electrons. If a neutral atom loses or gains one electron (or more), such as might happen during a chemical reaction, the resulting atom has an imbalance between the existing charges. This imbalance creates either an excess positive (+) charge, if the atom lost an electron, or an excess negative (-) charge, if the atom gained an electron. Atoms that have a charge are called ions, and they are very reactive and fundamentally important in chemical reactions.
Chemical Reactions: Ionic, Covalent, and Polar Covalent Bonds
Chemical reactions are important to all levels of biology. In the simplest terms, a reaction requires reactants and products. Reactants are the atoms or molecules that are involved with the change, and products are the resulting changed atoms or molecules. In most biological reactions, enzymes act as catalysts to increase the rate of a reaction. A chemical reaction occurs when reactants are joined together to create a product that has different chemical properties than the original reactants. This always involves an energy change and a change in the electron configuration around the original atoms. When electrons redistribute their orbitals to include two or more atomic nuclei, as is the case in a covalent bond, or donate or accept electrons, as is the case in an ionic bond, a chemical reaction has occurred. Two general types of bonds form during chemical reactions: ionic and covalent.
Ionic bonds form when the outermost, or valence, electrons of an atom are donated or received in association with a second atom. Because the electrons are now orbiting around the receiving atom and not their original atom, the receiving atom now has an imbalance between the number of protons and electrons and becomes a negatively charged ion. The donating atom also has a proton-electron imbalance and becomes a positively charged ion because it lost a negatively charged electron and the number of its protons remained the same. The resulting molecule has properties different from the original atoms. It is important to remember that because of the unequal electron distribution around the reacting atoms, the resulting ionic compounds have partial charges. This importance is developed in greater detail in Specialized Cell Structure and Function, but it explains the fact that water can dissolve any substance that has a partial charge on it. A typical example for an ionic bond is the joining of a sodium atom, which donates an electron, to a chlorine atom, which accepts the electron, to form sodium chloride, also known as table salt.
Covalent bonds occur when two or more atoms share their electrons. The electrons are not donated/accepted; instead, they incorporate their orbitals to create an electron cloud around all participating atoms. When the electrons are shared evenly around all reacting nuclei, there is no partial charge on the resulting molecule, as is the case when carbon covalently bonds with itself. However, in some cases, the electrons are not shared evenly and partial charges occur, as in the case of polar covalent bonds.
In reality, many bonds are actually a hybridization of ionic and covalent and have characteristics of both types. Atoms with polar covalent bonds share their electrons (covalent characteristic) unevenly (ionic characteristic), giving a slight positive (+) charge to one end of the molecule and a slight negative (-) charge to the other end. Water is a polar covalent molecule because the electrons spend more of their time around the oxygen atom because the oxygen atom has more protons acting as electron-magnets. Because of this uneven sharing of electrons, the oxygen end of the molecule has a slight negative charge, and the hydrogen end has a partial positive charge because the electrons are spending more time orbiting around the oxygen atom. The overall molecule has a partial positive and a partial negative end. As a result, water molecules tend to align themselves so that the positive end of one molecule aligns with the negative end of another molecule (opposites attract).
Notice also in the ionic model that the electrons are drawn away from the sending atom and accepted by the receiving atom. The covalent model shows the electrons being shared equally around all of the atoms, whereas the polar covalent shows the unequal sharing of the electrons.
In all cases, the driving force for any chemical reaction is a move toward greater stability of the atoms. To increase stability, atoms tend to react so that they lower their energy and increase their entropy (randomness or lack of organization). In chemical terms, this means that they seek to have a stable number of electrons in their outermost orbital. The stable number means that the outermost energy level is either completely full or completely empty. Chemists call this the v because often eight valence electrons are required to reach stability. Atoms react to achieve this electron configuration by donating/accepting electrons (ionic) or sharing them (covalent). Biomolecules are considered organic because they contain the element carbon and are covalently bonded.
Water
Water is one of the most unique molecules known to man and also one of the most important to biological systems. Not only does water exist in nature in all three states of matter (solid, liquid, gas), it also covers 75 percent of the earth and composes roughly 78 percent of the human body.
The uniqueness of water comes from its molecular structure. Because it is a polar covalent molecule, it has a slight positive and slight negative charge on opposite ends. Examine the illustration Water molecule and note two important characteristics. First, notice the location of the slight positive and negative ends. Second, observe that water is a bent molecule, not linear or straight.
Because water is a bent, partially polar molecule, it possesses the following biologically important characteristics of what is formed by the joining of many water molecules—all of them are critical to the creation and support of life on Earth:
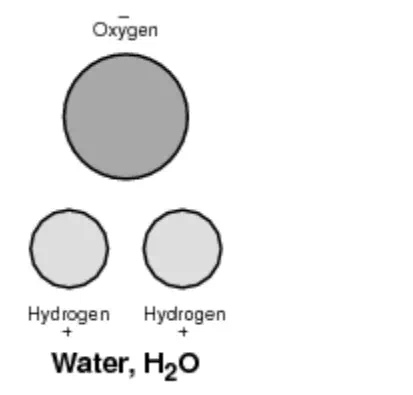
Water molecule.
- Polarity
- Hydrogen bonding
- Cohesion
- Surface tension
Polarity
Polarity simply means that the molecule has both a positively and negatively charged end. More important, the polarity of water is responsible for effectively dissolving other polar molecules, such as sugars and ionic compounds such as salt. Ionic compounds dissolve in water to form ions. This is important to remember because for most biological reactions to occur, the reactants must be dissolved in water. Because water is able to dissolve so many common substances, it is known as the universal solvent. Substances that cannot be dissolved by water (such as oils) are called fat soluble and are nonpolar, nonionic compounds that are strongly covalently bonded. Insoluble substances make excellent containers of water, such as cell membranes and cell walls.
Hydrogen Bonding
When water molecules align with each other, a weak bond is established between the negatively charged oxygen atom of one water molecule and the positively charged hydrogen atoms of a neighboring water molecule. The weak bond that often forms between hydrogen atoms and neighboring atoms is the hydrogen bond. Hydrogen bonds are very common in living organisms; for example, hydrogen bonds form between the bases of DNA to help hold the DNA chain together. Hydrogen bonds give water molecules two additional characteristics: cohesion and surface tension.
Cohesion
Because of the extensive hydrogen bonding in water, the molecules tend to stick to each other in a regular pattern. This phenomenon, called cohesion, is easily observed as you carefully overfill a glass with water and observe the water molecules holding together above the rim until gravity overtakes the hydrogen bonding and the water molecules spill down the side of the glass. Likewise, the cohesive property of water allows tall trees to bring water to their highest leaves from sources below ground.
Surface Tension
A special type of cohesion is surface tension. The tension on the surface of water occurs when water molecules on the outside of the system align and are held together by hydrogen bonding to create an effect similar to a net made of atoms. For example, the surface tension of water allows water spiders to literally walk on water.
Organic Chemistry
Carbon is an especially noteworthy element in living systems. The chemistry of carbon, organic chemistry, is a complete study unto itself. We will touch on several highlights that will be useful in succeeding sections.
The Uniqueness of Carbon
The carbon atom has four valence (outermost) electrons. Because of this unique configuration, it is easier for the carbon atom to share its four electrons with another atom or atoms than to lose or gain four electrons. Because each carbon is identical, they all have four valence electrons, so they can easily bond with other carbon atoms to form long chains or rings. In fact, a carbon atom can bond with another carbon atom two or three times to make double and triple covalent bonds between two carbon atoms. Long chains of carbon atoms with double and triple bonds are quite common in biology.
Carbon’s tendency toward covalent bonding with itself generates three unique characteristics that create a vast array of compounds, including those necessary to construct and support life:
Carbon to carbon (C-C) bonds form the backbone of all biomolecules and can include thousands of C-C bonds.
- The single bond that connects carbon atoms to carbon atoms is quite strong, so the subsequent long chains and ring structures are not fragile.
- The carbon-carbon covalent bonding pattern satisfies the Octet rule, making carbon compounds unwilling to react.
- Because carbon has four valence electrons and needs eight to satisfy the Octet rule, it can bond with up to four additional atoms, creating countless compound possibilities.
Functional Groups
In the simplest terms, the reactive part of any compound is called the functional group. Normally a functional group is a collection of atoms that operates as one reactive unit and is also the part of the molecule involved in a chemical reaction. Whereas carbon-to-carbon bonds are nonreactive, the instability of the functional groups drives chemical reactions that involve stable carbon-based compounds. For simplicity and reference, three functional groups are presented:
- Amine is identified by a central nitrogen atom that has three bonds, usually to hydrogen atoms. Amine groups form the basis for amino acids, which when bonded together form proteins.
- A Carboxylic group (COOH) is attached to the long carbon chains that form fatty acid molecules, which are a type of lipid lipids.
- Hydroxyl groups (OH) are very reactive. They are a component of alcohols, such as ethanol, the alcoholic component of adult beverages. The oxygen-hydrogen association is unique to this functional group and easily identifies it as an alcohol.
Dehydration Synthesis and Polymer Formation
Polymers are small molecules that can be bonded together to create larger molecules. Complex carbohydrates are made from small simple sugars joined together, and giant protein molecules are simply a series of smaller amino acid molecules bonded together. The prefix poly identifies this type of molecular addition. For instance, polysaccharides are large carbohydrates composed of multiple saccharide (sugar) units.
The chemical reaction that powers polymer formation is known by several names, including dehydration synthesis and condensation reaction. Regardless of the name, the molecules are joined by bonding sites created when a positively charged hydrogen ion (H+) is lost from one molecule and a negatively charged hydroxide (OH–) ion is lost from a neighboring molecule. The H+ and OH– combine to form water. So a dehydration synthesis joins two smaller units together with the loss of one water molecule.
Carbohydrates
Carbohydrates are organic compounds that are organized as ring structures and are always composed of the elements carbon, hydrogen, and oxygen. Carbohydrates are truly hydrates of carbon because the ratio of hydrogen atoms to oxygen atoms is always nearly 2:1, as in H2O.
They also have many functions. Most of the energy you receive comes from the carbohydrates that you eat. Plants make carbohydrates such as wheat, corn, and potatoes. Carbohydrates are normally consumed by animals either by eating the plant that manufactured it or by eating other animals. Humans also receive carbohydrates from whole grains, fruits, vegetables, milk, candy, soft drinks, and pasta.
Insects manufacture the carbohydrate chitin as a tough exoskeleton for protection, and lobsters and crabs use chitin for their shells. Finally, cellulose is probably the most widely used carbohydrate compound, comprising wood and wood products, such as paper.
Monosaccharides
The simplest biologically important carbohydrates are monosaccharides, meaning one sugar (mono = one, saccharide = sugar). The general formula for any carbohydrate is (CH2O)x where x is any number between three and eight. The most common monosaccharides (hexoses) are glucose, galactose, and fructose.
Glucose is the simplest monosaccharide and probably the most familiar sugar, especially if you have been in the hospital. In nature, glucose is the sugar that green plants produce during photosynthesis. It is also the main source of energy for cells. Medical procedures often require a glucose IV for recovering patients to regain their strength more quickly. Galactose is found in milk, and fructose gives fruit a sweet flavor. Although the chemical structure of each sugar differs, the chemical formula is the same: C6H12O6.
Disaccharides
Monosaccharides are joined together by dehydration synthesis to form disaccharides, or double sugars (di = two). The dehydration synthesis reaction releases water dehydration as a by-product. The most common disaccharide is sucrose, also known as table sugar, C12H22O11. Other common disaccharides include maltose (malt sugar) and lactose (milk sugar).
Polysaccharides
Further dehydration adds more sugar molecules together to form long chains known as polysaccharides. A polysaccharide generally refers to a carbohydrate polymer consisting of hundreds, even thousands of monosaccharides covalently bonded together. Cells use polysaccharides for a number of reasons, including the storage of excess glucose as starch in plants and glycogen in animals. The large polysaccharide cellulose is a structural component found in plants that gives them their rigidity and flexibility.
Lipids
Lipids are organic compounds that contain the same elements as carbohydrates: carbon, hydrogen, and oxygen. However, the hydrogen-to-oxygen ratio is always greater than 2:1. More important for biological systems, the carbon-to-hydrogen bonds are nonpolar covalent, which means that lipids are fat soluble and will not dissolve in water. There are four biologically important lipids:
- Fats
- Waxes
- Phospholipids
- Steroids
Fats
Fats are large molecules that are composed of three fatty acid molecules bonded to a glycerol molecule. The fatty acid molecule is a long chain of covalently bonded carbon atoms with nonpolar bonds to hydrogen atoms all along the carbon chain with a carboxyl group attached to one end. Because the carbon-hydrogen bonds are nonpolar, the chain is hydrophobic, meaning they are not water soluble. Glycerol is a three-carbon-chain compound that bonds with the fatty acids to create a fat. Typically, each carbon in the glycerol molecule bonds via dehydration synthesis to the first carbon atom from a fatty acid molecule so that the resulting fat molecule appears to have a glycerol head with three fatty acid chains streaming from it. This resulting molecule is called a triglyceride. Because carbon-hydrogen bonds are considered energy rich, fats store a lot of energy per unit. In fact, a gram of fat stores more than twice as much energy as a gram of a polysaccharide such as starch. Fats are lipids that are used by living organisms for stored energy.
A saturated fatty acid has hydrogen atoms bonded to all available carbon atoms. An unsaturated fatty acid has one or more carbon atoms double-bonded to the neighboring carbon atom so that fewer hydrogen atoms are needed to create a stable electron cloud. With fewer hydrogen atoms attached, the molecule is considered unsaturated with hydrogen atoms. So saturated fatty acids have more hydrogen atoms attached than unsaturated fatty acid chains. Through their metabolism, plants generally produce triglycerides that contain unsaturated fatty acids such as peanut oil or olive oil, whereas animals generally produce triglycerides that contain saturated fatty acids which humans sometimes convert into butter and lard.
Waxes
Waxes are similar to fats except that waxes are composed of only one long-chain fatty acid bonded to a long-chain alcohol group attached. Because of their long, nonpolar carbon chains, waxes are extremely hydrophobic (meaning they lack an affinity for water). Both plants and animals use this waterproofing characteristic as part of their composition. Plants most noticeably use waxes for a thin protective covering of stems and leaves to prevent water loss. Similarly, animals employ waxes for protective purposes; for instance, earwax in humans prevents foreign material from entering and possibly injuring the ear canal area.
Phospholipids
Phospholipids are similar to fats except they have two fatty acid chains bonded to a glycerol plus they contain the element phosphorus. Phospholipids are unique because they have a hydrophobic and a hydrophilic (water-soluble) end. Phospholipids are biologically important because they are the main structural components of cell membranes. The cell membrane is called a phospholipid bilayer because it consists of two phospholipid layers oriented so that the hydrophyllic “head” of both molecules face the exterior and the hydrophobic “tails” of both molecules create the interior of the membrane. Therefore, water and other cellular fluids are contained. The hydrophobic ends for both molecules face each other on the inside and allow for passage of acceptable, and some objectionable, materials through the cell membrane.
Steroids
Steroids are structurally different from the other lipids. The carbon skeleton of steroids is bent to form four fused rings that do not contain fatty acids. The most common steroid, cholesterol, is needed to make both the male (testosterone) and female (estrogen) sex hormones, and it is a component of cell membranes and is needed for the proper function of nerve cells. Excessive amounts of cholesterol, however, have been linked to heart disease. Another popular steroid group is the anabolic steroids that are man-made and mimic the effect of the male hormone, testosterone. Originally intended as a treatment for anemia and certain diseases that destroy muscle, athletes have recently been using them to increase muscle mass, stamina, and strength—which they will do. However, the performance-enhancement drugs come with a price. The anabolic steroids are linked to increased cholesterol levels, mood swings, reduced sex drive, possible infertility, and possible connections between liver damage and the resulting liver cancer. Certain beneficial fat-soluble hormones, such as cortisol, are also familiar steroids.
Proteins
Proteins are organic compounds that contain the element nitrogen as well as carbon, hydrogen, and oxygen. Proteins are the most diverse group of biologically important substances and are often considered to be the central compound necessary for life. In fact, the translation from the Greek root word means “first place.” Skin and muscles are composed of proteins; antibodies and enzymes are proteins; some hormones are proteins; and some proteins are involved with digestion, respiration, reproduction, and even normal vision, just to mention a few.
Amino Acids
There are obviously many types of proteins, but they are all made from amino acidsbonded together by the dehydration synthesis. By continually adding amino acids, called peptides, two amino acids join together to form dipeptides; as more peptides join together, they form polypeptides. Proteins vary in length and complexity based on the number and type of amino acids that compose the chain. There are about 20 different amino acids, each with a different chemical structure and characteristics; for instance, some are polar, others are nonpolar. The final protein structure is dependent upon the amino acids that compose it. Protein function is directly related to the structure of that protein. A protein’s specific shape determines its function. If the three-dimensional structure of the protein is altered because of a change in the structure of the amino acids, the protein becomes denatured and does not perform its function as expected.
Protein Structure
The three-dimensional geometry of a protein molecule is so important to its function that four levels of structure are used to describe a protein. The first level, or primary structure, is the linear sequence of amino acids that creates the peptide chain. In the secondary structure, hydrogen bonding between different amino acids creates a three-dimensional geometry like an alpha helix or pleated sheet. An alpha helix is simply a spiral or coiled molecule, whereas a pleated sheet looks like a ribbon with regular peaks and valleys as part of the fabric. The tertiary structure describes the overall shape of the protein. Most tertiary structures are either globular or fibrous. Generally, nonstructural proteins such as enzymes are globular, which means they look spherical. The enzyme amylase is a good example of a globular protein. Structural proteins are typically long and thin, and hence the name, fibrous. Quaternary structures describe the protein’s appearance when a protein is composed of two or more polypeptide chains. Often the polypeptide chains will hydrogen bond with each other in unique patterns to create the desired protein configuration.
Enzymes
Most enzymes are proteins and therefore their function is specific to their structure. Enzymes function as a catalyst to increase the rate of virtually all the chemical reactions that take place in a living system. The enzymes, like all catalysts, are not consumed but are constantly reused to catalyze the same specific reaction. Enzymes depend on the correct structural alignment and orientation at the active site of the protein and the appropriate site of the reactants, or substrate, before the reaction can proceed. This geometric interaction between the enzyme and the substrate is referred to as the “lock-and-key model” because the enzyme’s action parallels the action of a lock into which is fitted the key (substrate). If the key and lock do not match, the action does not work.
It is the same with enzymes and substrates. The active site for the enzyme and the appropriately matched site of the substrate must physically join before the reaction can occur. That is why the structure of the enzyme is so important. The enzyme binds with the appropriate substrate only in the correct alignment and orientation to connect the molecules. The resulting enzyme-substrate complex enables the reaction to occur. Finally, the products are formed and the enzyme is released to catalyze the same reaction for another substrate of the same type of molecule. Enzymes may fail to function if they are denatured. Remember the model simplifies your understanding of the process; in reality they are three-dimensional molecules.
Hormones
Hormones are chemical messengers produced in one part of the body to function in a different part of the body. Although fat-soluble hormones are made from steroids, water-soluble hormones such as the growth hormone are made from amino acids. Hormones function similarly to enzymes in that both require a specific receptor and perform a specific function. After a hormone is created and secreted by a cell, it travels—usually via the bloodstream—to its target cell. The target cell is the point of action that the hormone recognizes, binds to, and thereby delivers the chemical message. The hormone identifies the target cell by its receptor protein and employs the same lock-and-key process.
Nucleic Acids
Nucleic acids, which are composed of nucleotides, are very large and complex organic molecules that contain the genetic code for that organism. Two closely related types are needed to transmit the genetic information from parent to offspring: DNA and RNA. Not surprisingly, they also share structural similarities.
Nucleotides
Both DNA (deoxyribonucleic acid) and RNA (ribonucleic acid) are polymers of individual nucleotides. Each nucleotide has three components:
- Five-carbon-ring sugar (deoxyribose or ribose)
- Phosphate group
- Nitrogen base
Both DNA and RNA have four nitrogen bases available to construct nucleotides. Three of the nitrogen bases are the same. Review the following table for similarities and differences between DNA and RNA.
Nitrogen Bases | |
DNA | RNA |
Adenine | Adenine |
Thymine | Uracil |
Cytosine | Cytosine |
Guanine | Guanine |
Both DNA and RNA are large molecules of successive nucleotides bonded to the main chain by the dehydration synthesis reaction. Additional nucleotides connect when the phosphate of one nucleotide bonds with the nitrogen base of the next nucleotide.
DNA
Francis Watson and James Crick discovered the structure of DNA, which today we refer to as a double helix. The DNA double helix is actually two complementary strands of DNA that wrap around each other and are held in place by hydrogen bonding between the two strands. The resulting structure is a spiraling geometric molecule that looks like a twisted ladder, a.k.a. a double helix. It is important to remember that DNA molecules are so large they cannot escape the nuclear membrane that surrounds the nucleus of a cell.
Each DNA molecule consists of many genes. Each gene is composed of hundreds or usually thousands of nucleotides arranged in a specific order. The unique sequencing of the nitrogenous bases associated with the nucleotides dictates the primary structure of the protein to be created by the cell. Your chromosomes and those of all living creatures consist of long strands of DNA that store the heredity information, or genetic code, for that organism.
RNA
RNA (ribonucleic acid) is similar to DNA in that both are composed of long chains of nucleotides. However, RNA is a single strand and therefore much smaller than DNA. Although DNA contains the genetic information that determines all cell characteristics and functions, RNA stores and transfers the genetic code contained in the DNA. Its smaller size allows RNA to move freely through the nuclear membrane to transport the genetic instructions, usually to the ribosomes, for protein assembly.
Vitamins and Minerals
Vitamins are organic compounds that are required by advanced animals in small amounts on a regular basis. Like essential amino acids, there are 13 vitamins that are required but not produced by the human system. Unlike essential amino acids, these vitamins are required in minute quantities. If deficient or in excess, certain maladies occur in humans, such as beriberi, anemia, rickets, and skin lesions. In general, vitamins are coenzymes, or parts of enzymes, that function to assist a specific enzyme to catalyze (increase the rate of) a reaction. Some vitamins are fat soluble and others are water soluble.
Fat-soluble vitamins are probably the most common vitamins for some people. Unlike water-soluble vitamins, they remain stored in the fat deposits of a body for long periods of time and may accumulate to overdose levels. Note that the only vitamin humans can make is Vitamin D. Vitamin D is made when cholesterol is acted upon by enzymes and sunlight. It should also be noted that the fat-soluble Vitamin K is produced in small quantities in the human intestine by the action of mutually beneficial intestinal bacteria.
Water-soluble vitamins generally function within the cell to help catalyze cellular reactions such as cellular respiration. For your reference, cellular respiration is the process of harvesting energy from the breakdown of food molecules that takes place inside individual cells. Unlike fat-soluble vitamins, excess water-soluble vitamins do not remain stored in the body, but are excreted in urine and feces. Water-soluble vitamins include the eight different types of B complex vitamins and Vitamin C.
Minerals are naturally occurring inorganic substances required in trace amounts for normal body functions such as the development of strong bones and teeth, proper muscle and nerve functions, and construction of red blood cells. Like vitamins, these essential minerals are not produced by humans, so they must be consumed on a regular basis. Because they are water soluble, excessive amounts are eliminated through normal urinary functions and perspiration.
Comments are closed.